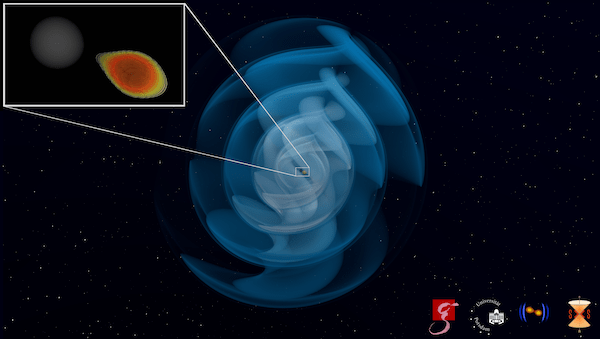
Still image from a numerical simulation of a black hole / neutron star merger. Image credit: S.V.Chaurasia (Stockholm University), T. Dietrich (Potsdam University and Max Planck Institute for Gravitational Physics), N. Fischer, S. Ossokine, H. Pfeiffer (Max Planck Institute for Gravitational Physics), ,https://www.ligo.org/detections/NSBH2020/files/BHNS_GW200115.png.
Scientists have long predicted that neutron stars and black holes could orbit each other and collide, but they had never observed it, until last January! Ten days later, they found another one!
The LIGO/VIRGO collaboration (LVC) studies gravitational waves, a type of information fundamentally different from electromagnetic light. When massive objects, like black holes and neutron stars, collide, they emit waves that stretch and squeeze the very fabric of spacetime. We can observe these events by very, very carefully measuring the distance between two perpendicular detector arms. If optical telescopes are our eyes on the universe, gravitational waves are like our ears, providing new kinds of data that allows us to study the universe in novel ways.
Since 2015, the LVC has detected plenty of binary black holes and at least one binary neutron star, but they’ve never observed a pair with one of each. Black holes and neutron stars are both compact objects that form when stars reach the ends of their lifetimes and collapse, but the two arise from different types of stars. There’s no physical reason that we shouldn’t get compact binaries with one of each type, but they’re thought to be relatively rare systems. With the two events observed in January 2020, scientists can now confidently say these systems exist, and can begin studying their properties. The graph below shows time versus frequency for the first of the two events in the Livingston detector. Though it’s faint, there’s the distinctive “chirp” of a binary merger: a curved line that rises in frequency across the plot.

Gravitational-wave “chirp” of GW200105 in time-frequency space. Image credit: Fig. 1 in the paper.
Using a set of complicated methods to model the detector noise and GW signal, they determined that the first pair of objects were about 9 and 1.9 times the mass of the sun, and the second pair were 6 and 1.5 solar masses. For both of these, the larger object is consistent with a black hole, and the smaller object is consistent with a neutron star. The statements must be qualified with “consistent with” since scientists cannot definitively identify the sources, for reasons I’ll explain later. The following plot shows the component masses. Since they cannot be measured perfectly, they are shown using posterior probability distributions that give a range of values that fit the data.

Component masses for the two mergers in solar masses, shown using the posterior distributions. On the right, the green distributions show the theoretical maximum neutron star mass from two models. Image credit: Fig. 4 in the paper.
These observations help fill out the “stellar graveyard”: the known population of neutron stars and black holes. The graph below depicts this population. These events, the ones highlighted in the middle, are the first of their kind, making them exciting additions to scientists’ catalog!
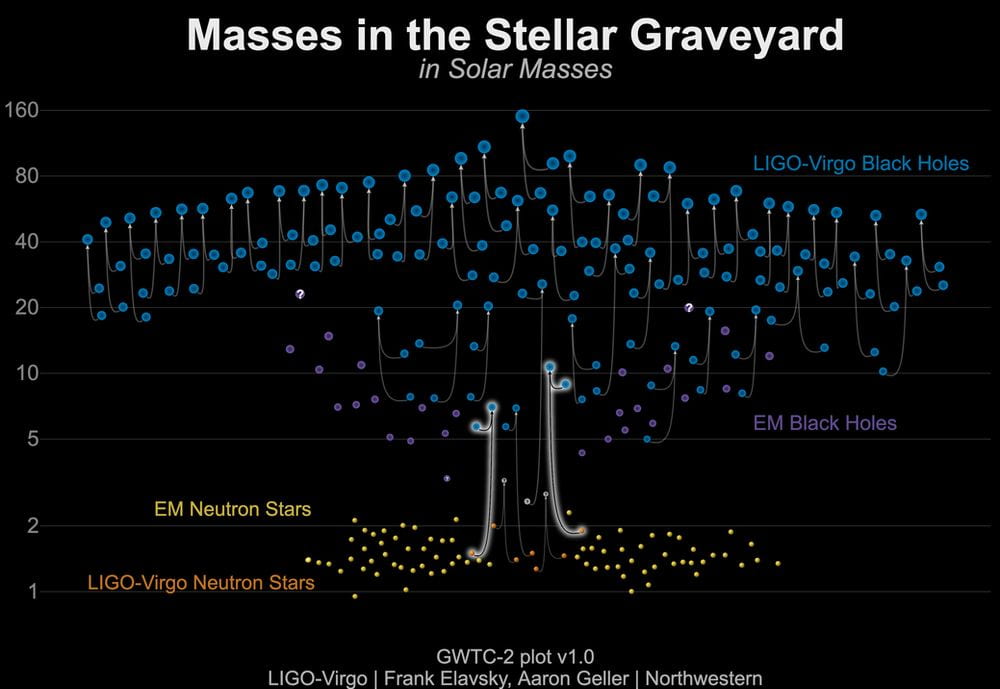
Known black holes and neutron stars with the two recently published mergers highlighted. Image credit: LIGO-Virgo/Northwestern U./Frank Elavsky & Aaron Geller, https://www.ligo.org/detections/NSBH2020/files/nsbh-graveyard.png.
When black holes collide, there’s nothing there that could emit light. Binary neutron stars, on the other hand, produce a gigantic explosion called a kilonova. Scientists were lucky enough to see one of these, scouring an area of the sky 150 times the size of the moon, after LIGO reported a neutron star merger in 2017. With the two NSBH events in January 2020, no light counterparts were found. The sky localization was large, so telescopes weren’t able to search the entire area, and the systems were very far away, so any signal would be very faint. Regardless, depending on how the black hole swallows the neutron star, there might not be any extra light at all. Since there was no light detected, though, LIGO cannot definitively say the smaller objects were neutron stars, but rather classify them based on their masses.
From these observations, scientists can start to empirically test formation scenarios for neutron star-black hole systems. Future detections could help constrain the Hubble constant—a fundamental parameter of the universe—and population properties of the universe’s black holes and neutron stars. Gravitational-wave astronomy is still in its early years, so as new detectors are built and the current ones upgraded over the next decade, expect many more exciting discoveries about some of the most enigmatic objects in the universe!
References:
https://arxiv.org/pdf/2106.15163.pdf
You must be logged in to post a comment.